Currently existing engineering materials do not have the capability to perform under the operational conditions of future fusion power reactors, which correspond to intense levels of high energy neutron irradiation and high heat loads. Thus, innovative technologies have to be implemented for the development of new materials, able of withstanding the high neutron irradiation levels and temperatures of a fusion reactor.
Candidate structural materials for fusion energy systems are ferritic/martensitic (FM) Fe-Cr based steels. In their present development state, these materials are not capable of withstanding the extreme conditions of fusion reactors. However, the optimization of FM steels and the development of new classes of radiation resistant materials are held back by the limited knowledge of the underlying mechanisms, which lead to their deterioration under irradiation. Therefore, the relevant processes need to be understood from atomistic to mesoscopic level.
Fe+ ion irradiation effects of Fe-Cr alloys
For the understanding of radiation damage in Fe-Cr steels, fission reactors are utilised for the irradiation of these materials. However, the fission neutron energy spectra differ considerably from those of fusion reactors and thus the extrapolation of the observed results to a fusion-relevant environment is unreliable. This shortcoming can be remedied by employing Fe ion irradiation instead of fission neutrons. Since the major damage in a material is caused by the primary knock-on atoms (PKA), appropriate Fe ion energies can be used to imitate the PKA damage produced by fusion neutrons. Further, the capability of employing different energies of ions and different ion species or combination of them make ion irradiation a valuable tool for understanding the fundamental aspects of radiation damage induced in a fusion irradiation environment and it can provide a useful data base for verification of the fusion material models
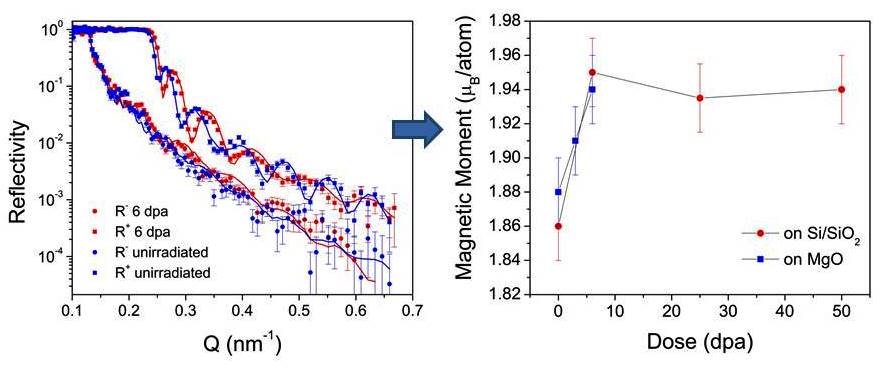
Left: Polarised neutron reflectivity (PNR) spectra of unirradiated and 500 keV Fe+ ion irradiated to 6 displacements per atom (dpa) at room temperature Fe-10at%Cr films grown on different substrates. Right: The magnetic moment versus dose at room temperature determined from least square fittings of the PNR spectra.
Our previous studies of Fe+ ion irradiation effects on polycrystalline Fe films showed significant changes in the structural and magnetic properties of iron. Currently, our work is focused on the Fe+ ion irradiation effects on Fe-Cr polycrystalline films as a function of irradiation dose and temperature, dose rate, Cr content and Fe+ ion energy. Well-established techniques are available for the fabrication and characterization of such materials including X-ray and neutron scattering techniques and positron annihilation spectroscopy among others.
Studies of Irradiation Defects
Fig. 2. Resistivity recovery rate of Fe-10%Cr alloys, un-doped and doped with C as a function of annealing temperature after 5 MeV proton irradiation at 50 K. The spectra are recorded during gradual post-irradiation annealing, which is performed in-situ within the IR2 facility. The visible influence of carbon on features II and IV of the spectra reveal interactions of irradiation defects with the carbon impurities.
Irradiation of materials by energetic particles causes displacement of atoms from their lattice positions and results in the creation of large numbers of sub-nanometer sized defects (mainly point defects). The accumulation of such defects gradually alters the microstructure and leads to the degradation of material properties through mechanisms like hardening and embrittlement, phase instabilities, solute segregation etc. The study of point defects is challenging due to their small size, which makes them invisible even to the highest resolution imaging methods as, e.g., transmission electron microscopy. Furthermore, they are highly mobile at typical operating temperatures of structural materials and thus they almost instantly disappear through interaction with other defects or solutes. For these reasons point defect studies employ indirect experimental techniques as electrical resistivity, positron annihilation spectroscopy, diffuse X-ray scattering and others. Measurements have to be performed at cryogenic temperatures in order to reduce the mobility of defects.
To address these problems one of the main research activities of FTG is the development and application of experimental techniques for the study of primary radiation defects in fusion materials. In this context, the specialized irradiation facility IR2 has been commissioned at the TANDEM accelerator of NCSR "Demokritos" and other techniques such as, e.g., Positron Annihilation Spectroscopy, are being developed.